|
|
|
|
Sub- and superradiance of
matter-wave quantum emitters. We realized
cooperative radiative dynamics
in ar rays of matter-wave
quantum emitters, in which the
coupling to a common radiative
environment enhances, suppresses, or even
qualitatively changes the
nature of spontaneous
emission. Usually, the distance between
quantum emitters is small
compared to the coherence
length of the readiation, such
that direct contributions
of the rapidly escaping
photons are negligible. In
our platform
(see spontaneous
emission of
matter waves,
below), the
carriers of
interactions
are not
photons but
free atoms,
which have
wavelengths
comparable to
those of
photons but
are 10 orders
of magnitude
slower. This
allows access
to novel, and
hitherto
inaccessible,
aspects of Dicke physics. By
initializing our emitter
arrays with atomic excitations
in superfluid and Mott
insulating states, we were
able to observe near-perfect
subradiance and directional
superradiance, examine
non-Markovian effects arising from
propagation delays, and directly detect the
formation of coherence across
the quantum emitters.
By
merging concepts of condensed
matter physics, atomic
physics, and quantum optics, our work provides
both fundamental insights and
novel connections between
fields. Article: [Nat.Phys.]
[SharedIt]
* Press: [SBUNews][PHYS.ORG][AZoQuantum|uk][Enerzine|fr][FOCUS|pl][MuyInteresante|es(transl)][OkDiario|es(transl)][FisikHaber|tr] [weixin|cn(transl)]
[SOHU.com|cn]
[HelloDD|kr(transl)]
[ubu|es(transl)]
The study of
collective radiative phenomena
was pioneered by R. H. Dicke in
1 954, but the
question of how to keep
track of non-Markovian effects
due to a combination of
propagation delays and strong
coupling remains largely a
mystery. In related theoretical
work, we showed that a
minimal scenario consisting of
two fully excited quantum
emitters decaying into a one-dimensional
single-band waveguide -
sufficient to break the
assumption of independence
between photons and to go beyond
Dicke s formalism - is exactly
solvable, revealing decay
behavior that evades approximate
and numerical methods. Our
findings open up an analytical
pathway for scenarios in which
distant quantum emitters are
strongly coupled to
one-dimensional waveguides. Article:
[Phys.Rev.Res.]
* Press:
[SBUNews][PHYS.ORG][AZoQuantum|uk][Enerzine|fr][FOCUS|pl][MuyInteresante|es(transl)] [OkDiario|es(transl)]
[FisikHaber|tr] [weixin|cn(transl)]
[SOHU.com|cn]
[ubu|es(transl)]
Formation of matter-wave polaritons. We realized
interacting polaritonic
quasiparticles in a lattice and investigated their behavior
across the superfluid-to-Mott
transition. The
observed behavior is consistent
with a combination of the Bose-Hubbard
and Weisskopf-Wigner models. A
matter-wave polariton can be
visualized as a hopping atom
surrounded by a cloud of
matter-wave excitations.
Analogous features are known
from the formation of exciton
polaritons, where excitons are
dyamically bound to guided photons.
The coupling hybridizes the
dispersion relation of the heavier
constituent with that of the
lighter one, giving rise to renormalized
transport and interaction
properties. In our system, we prepared
the lower polariton branch
dominated by the heavier constituent
(the atom hopping in the
lattice). We directly detected the
presence of polaritons by
probing their excitation
spectrum and found
very good agreement of the
inferred hopping with our theoretical
expectations. In
contrast to their photonic
counterparts, matter-wave
polaritons are dissipation-free
and tunable and thus can serve
as an ideal testbed for
polariton physics. Article: [Nat.Phys.] [SharedIt] Press: [SBUNews]
[AAU]
[PHYS.ORG(feature)]
[N+1|ru
(transl)]
In
preceding theoretical
work,
we calculated the
details of the
polaritonic band
structure based
on
a ormalism that
captures
generalized
situations in
which both
the radiation and the
matter-wave
excitations
in the lattice are
subject to lattice
transport.
The resulting band
structure of the
matter-wave
polaritons is
exotic and cannot
be obtained by
from a simple
periodic
potential.
With the
flexibility to
tune the
parameters, it
becomes possible
to realize a wider
platform for studying
low-dimensional
frustrated
systems. Article:
[Phys.Rev.A]
Spontaneous emission
in a structured vacuum. We
studied the dynamics of matter-wave quantum emitters
coupled t o the band structure of an optical lattice.
Contrary to free-space decay (which provides an analog
to optical decay near a single band edge), the
dynamics in a band, which is bounded by two edges, can
change from exponential decay to fully coherent
oscillations. We investigated the interplay between
the emitters' excitation energy, the vacuum coupling,
and the bandwidth of the lattice, and characterized
the two contributing bound states of the matter-wave
radiation field. The understanding how quantum emitters interact with
the modified vacuum is at the heart of waveguide
QED, one of the frontiers in quantum optics, and
this concerns in particular the emergence and
properties of atom-photon bound states in photonic
crystals. Our experiment sheds light on the
link between coherent emitter dynamics, fractional
decay, and the ability of such bound states to
interfere.
Article:[Phys.Rev.Res.]
In follow-up theoretical work, we generalized
our earlier single-emitter model to emitter arrays with a single excitation and
radiative coupling to a full band structure. Our
analytic model successfully reproduces the observed
momentum distributions for emission into free
space well as into the bands of an attractive and repulsive
lattice potential, corresponding to co-located and
shifted wells. Moreover, the model fully captures the
observed decay in the crossover regime, reproducing
propagation effects and reabsorption by neighboring
emitters that lead to pronounced oscillations at long
times
Article: [Phys.Rev.A]
Spontaneous emission of matter waves. We
demonstrated the spontaneous emission of matter
waves from
a tunable open
quantum system. The quantitative
understanding of spontaneous emission harks back to
the early days of quantum electrodynamivcs (QED),
when in 1930 Weisskopf and Wigner, using Dirac's
radiation theory, calculated the transition rate of
an excited atom undergoing radiative decay. Their
model, which
describes the emission of a photon through coherent
coupling of the atom's dipole moment to the
continuum of vacuum modes, reflects the view that
spontaneous
emission into free space, driven by vacuum
fluctuations, is inherently irreversible. We
realized the Weisskopf-Wigner model in a novel
context that allowed us to go beyond the model's usual
assumptions. For this purpose, we created
microscopic atom traps in an optical lattice acting
as tunable matter-wave quantum emitters --
artificial emitters that spontaneously decay by
emitting single atoms, rather than single photons,
into the surrounding vacuum. Our ultracold system,
which provides a matter-wave analog of photon
emission in a photonic-bandgap material, revealed strong-coupling
behavior beyond standard exponential decay with its
associated Lamb shift. It includes partial backflow
of radiation into the emitter, and the formation of
a long-predicted, exotic bound state in which the
emitted particle hovers around the emitter in an
evanscent wave. - Our system provides a flexible
experimental platform for simulating open-system
quantum electrodynamics and for studying dissipative
many-body physics with ultracold atoms. [further
details] Article: [Nature]
[SharedIt] * Press:
[NatureN&V]
[pro-physik.de]
[SBUNews]
[Gizmodo]
[Investigacion
y Sciencia|es]
In preceding theoretical work, we used the Weisskopf-Wigner formalism to
revisit a proposal by the group of J.I.Cirac
at MPQ
and determine the details of the
(non-)Markovian decay and emission dynamics considering an isolated matter-wave
emitter in our waveguide geometry. In our work, we
also calculated the spatial structure of the bound
state for negative excitation energy, which is in excellent agreement with our
measurements. [further
details] Article: [Phys.Rev.A]
***
|
|
|
|
Diffracting
matter waves without a potential. We explored a
novel regime of diffraction arising in the dynamical
response of an atomic Bose-Einstein condensate coupled
to a optical standing wave. Throughout the long history
of diffraction, it has generally been taken for granted
that the diffracting object can be described through a
(position-dependent) potential with absorptive or
dispersive character. This
includes diffraction from material structures and
gratings, ponderomotive potentials, as well as
Kapitza-Dirac diffraction of atoms from a standing wave
of light. The latter can be described via an
optical potential, and is one of the standard tools in
atom optics and metrology. We demonstrated that
Kapitza-Dirac diffraction (i.e. the diffraction of
matter from light) can in fact occur without a potential
being present. In our experiments, we studied the
dynamics of a condensate after switching on a resonant
driving to the orbitals of a deep, state-selective
optical lattice. We observed diffractive dynamics
that remains coupled to coherent oscillations of the
internal degree of freedom, violating the usual
adiabatic (Born-Oppenheimer) approximation needed for
the introduction of an optical potential. This
represents a hitherto unexplored regime in which the
dressed states undergoing
diffraction are strongly mixed by zero-point motion. Our
study sheds new light on the role of potentials in
diffraction, and on the nature of dressed states arising
in Rabi-type dynamics. We explored its utility for atom
interferometry and future realization of dissipative
quantum systems [further
details].
Article: [Phys.Rev.A]
|
|
|
|
Abating
the effects of disorder with weak interactions.
In studies of the dynamics of a Bose-Einstein co ndensate in a tilted
incommensurate optical lattice, we demonstrated a
nontrivial interplay between interactions and
disorder. When a weak constant force is applied to
atoms moving in a lattice potential, they
undergo periodic motion known as Bloch oscillations
(rather than accelerating as free particles). It is
well established that for condensates these
oscillations damp out due to either the presence of
disorder or collisional interactions. However, we
observed that when interactions and disorder are
present simultaneously, they can compete with each
other such that more interactions actually mean
less, rather than more, damping. This screening
effect vanishes once the interactions become
stronger than the disorder (strong interactions
cooperate with disorder, cf. eg. our earlier work).
Our results are of relevance for the description of
systems ranging from superfluid helium in porous
materials to superconductivity in granular or
disordered materials. [further
details]
|
|
|
Crossing the quantum-to-classical boundary
with a coupled-rotor system The
understanding of how class ical dynamics
can emerge in closed quantum systems is a problem of
fundamental importance. Remarkably, while classical
behavior usually arises from coupling to thermal
fluctuations or random spectral noise, it may also be
an innate property of certain isolated, periodically
driven quantum systems. By exposing a Bose-Einstein
condensate to a periodically-flashed, two-color
standing wave of light, we have for the
first time realized a system of two coupled, kicked
quantum rotors, and have
discovered a quantum-to-classical
transition. The simple quantum kicked rotor,
which one obtains when using a single-color standing
wave only, is a well-studied paradigm system for
quantum chaos. Here, unless very specific conditions
are met, the atomic velocities remain frozen in time
due to quantum interference, despite continuous
periodic kicking. However, already when just two such
off-resonantly kicked rotors are coupled, new physics
can appear. In our work, we found that the ability of
each rotor to freeze the atoms' velocities can be
destroyed, and that the coupling between the two
rotors instead produces classical momentum diffusion.
Our work sheds light on the fundamental question how
the quantum and classical worlds are connected. [further
details] Article:[Phys.Rev.Lett.]
* Press:[PHYS.ORG(feature)]
|
|
Probing
an ultracold-atom crystal using atomic matter waves.
We explored the scattering of atomic matter waves from
ultracold atoms confined in an optical lattice. By
shining a one-dimensional Bose
gas (probe) onto a Mott insulator (target), we
observed Bragg diffraction peaks that reveal the
spatial ordering and localization of atoms on
individual lattice sites. Our in-situ interrogation
technique is non-destructive, and it precludes
limitations on spatial resolution since the de Boglie
wavelength of the incident
atoms can be tuned freely. For weak confinement, we
observed inelastic excitations of atoms in the target,
which connect to a quantum "Newton's cradle" in the
free-atom limit. Furthermore, we used atomic de
Broglie waves to detect forced antiferromagnetic
ordering in an atomic spin mixture, demonstrating the
suitability of our method for the non-destructive
detection of spin-ordered phases in strongly
correlated atomic gases. Our work extends matter-wave
diffraction, a technique that
has already proven useful in various scientific
disciplines, to the realm of ultracold quantum matter.
Our method is similar to the diffraction of neutrons
for the characterization of solid-state systems, but
at energies that are a billion times lower. It also
provides a nice example of wave-particle duality,
where ultracold atoms serve both as localized
particles and as coherent waves diffracting from them.
[further
details] * Article: [Nat.Phys.]
* Press:[PHYS.ORG]
|
|
Impurities make an
ultracold-atom crystal behave like an amorphous
glass. We studied one-dimensio nal
gases of bosonic atoms subject to localized atomic
impurities and observed behavior consistent
with an exotic quantum phase called a Bose glass.
Two-species mixtures of quantum gases
can form so-called "quantum emulsions" (see next
paragraph). When one of the two species is pinned in
place, its frozen emulsion pattern can effectively act
as a static disorder potential for the more
mobile species. It is predicted that such atomic
impurities can cause a disorder-driven quantum phase
transition from a perfectly-ordered Mott insulator
crystal to an amorphous Bose glass. In our
experiment, we created "frozen" atomic impurities by
loading the atoms of one species onto
the sites of a deep, species-specific optical lattice.
We found that the other, more mobile species is driven
to a localized, insulating state with a flat
excitation profile, consistent with the expectation
for a Bose glass. One key motivation for the use of atomic
impurity disorder, as opposed to the standard
optical disorder potentials, is the difference in
spatial correlation properties. Indeed, we found that
using uncorrelated atomic disorder much more easily
drives the system into the amorphous phase. [further details]
* Article: [Phys.Rev.Lett.]
|
|
|
Poster gallery
|
|
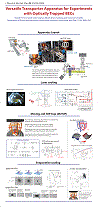
|
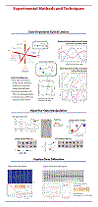
|
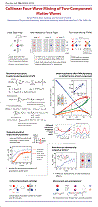
|
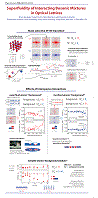
|
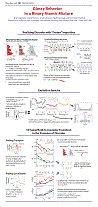
|
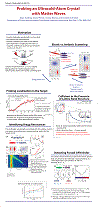
|
|
|
|
|
|
|
|
|
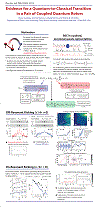
|
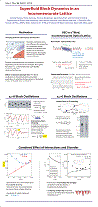
|
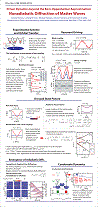
|

|
|
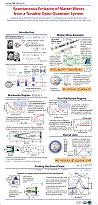
|
|
|
|
|
|
|
|
|
|
|
|
|
|
|
 |
|